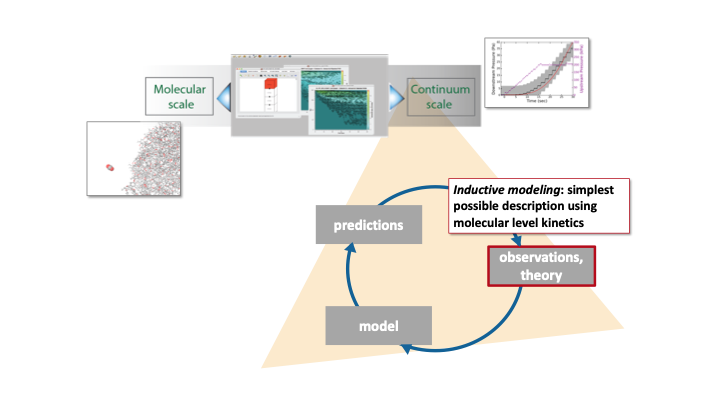
Our research focuses on understanding how complex reacting systems work across extended time (femtoseconds to days) and length (nanometers to centimeters) scales using coupled reaction-diffusion simulations. We discover mechanisms inductively, rather than applying models deterministically as would be done using known physics and chemistry. This allows us to construct models that are physically accurate and predictive, and that give us detailed insights to the workings of systems. We are especially interested in complex spatially distributed reacting systems, which are challenging to describe and understand. Our goal is to connect atomic scale processes to observations quantitatively, and to discover some of the essential mechanisms at play.
At Lawrence Berkeley National Lab, we are currently studying the stochastics of infrequent events in solution, thin films and catalytic interfaces, and how they influence durability in solar fuels systems, solar-driven charge injection and photocatalysis, catalytic cascades, and imaging precision in photoresists. We are also working on experimental designs for studies of ultrafast protein reactions. Past work has included work on water evaporation, chemical transformations in aerosol, and simulations of interfacial and thin film processes such as temperature programmed desorption, and chemical vapor deposition of metals and semiconductors. Although these are very diverse types of systems, they share important mechanistic elements, allowing us to see patterns across nature and understand fundamentals of reactivity in liquids and solids, and at their interfaces.
In all cases, the modeling work is closely integrated with experimental studies, which provide information on elementary reaction and transport processes and composition and structure as a function of time. The data are essential for both construction of the models, and validation of their simulation results. Models constructed this way are physically realistic and predictive, and provide a lot of information on the evolution of the reacting system that is often not experimentally accessible.
We use stochastic simulation methods, which are a type of kinetic Monte Carlo calculation and are well-suited to simulations of systems spanning wide length and time scales. The simulations perform a random walk through a set of probability-weighted events, and predict spatially resolved composition vs time maps that can be analyzed to predict experimental observations. When elementary rate constants are used, the simulations generate an absolute time base that allows direct comparison between simulated and measured data. Our code, Kinetiscope, is freely available in open access along with tutorials and sample simulations.