We are interested in learning how complex reacting systems work, and through this work see that there are interesting patterns across nature. Understanding these patterns can help us build models for new systems by re-using model framework elements and results for one system to gain new insights to others that have similar mechanistic elements.
Some of the elements we are finding to be important include uptake and release of molecules across gas-liquid, liquid-solid and gas-solid phase boundaries, diffusion through nanoscale channels, complex oxidation-reduction reaction networks, and influences of continuously changing environments on reactivity.
We thank the Department of Energy Basic Energy Sciences and the National Institutes of Health for funding this work.
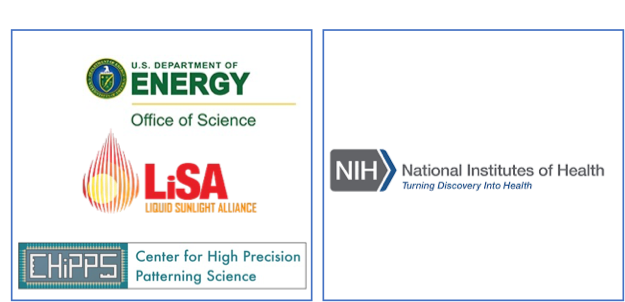
I. Key processes involved in solar-to-chemical energy conversion
1. Water oxidation (DOE Office of Science program)
The oxygen evolution reaction (OER) is known to be a bottleneck in the process of photocatalytic water splitting, hindering the overall process. We are investigating the kinetic factors that control its rate under sunlight in order to guide the design of future catalysts. These focus especially on charge carrier (electron and hole) formation and transport in a reacting system.

Photo-driven water oxidation catalysis: rutile TiO2 (110) surface
Team: Pan Wang, Gabriel Benitez (LBNL), Dunwei Wang, Tianying Liu (Boston College)
Experimental studies have shown that the rate of light-driven OER on metal oxides has a high order dependence on photohole concentration over a range of light intensities. We are performing simulation studies to connect these observations to detailed theoretical studies of the catalytic process on a TiO2 surface. The calculations are designed to examine the underlying chemistry and physics of the reaction, and reveal how hole kinetics influence it. In particular, we are interested in the interplay between hole generation, loss and transport, and the multistep catalytic cycles involving Ti and O sites on the catalyst surface. We have examined two cases involving the same free energy landscape but treating hole kinetics differently (holes can or cannot diffuse after they are trapped at the surface). The results are showing that the nature of the interplay between hole transport and reactions changes dramatically from low to high light intensity, and that thermal steps such as O-O coupling and proton losses can be more important for controlling the overall OER rate than the photochemical steps. Temperature effects are currently under study.
Photoanodes for Dye-sensitized photoelectrochemical synthesis cells
Diad modeling team: Ramzi Massad, Thomas P. Cheshire, Chenqi Fan
Dye photophysics team: Thomas P. Cheshire, Jéa Boodry (LBNL), Erin A. Kober, M. Kyle Brennaman, Paul G. Giokas, David F. Zigler, Andrew M. Moran, John M. Papanikolas, Gerald J. Meyer, Thomas J. Meyer (U. North Carolina)
We have investigated water oxidation by dye-catalyst diads attached to TiO2 under continuous natural sunlight. The question under investigation was whether the slow OER kinetics of the diad were due to sparse solar photons and charge carrier losses due to back electron transfer from the semiconductor to the adsorbed molecules. The kinetics of dye photoexcitations were established by quantitative modeling of experimental ultrafast transient pump-probe measurements, and converted to a continuous illumination mechanism. The simulations showed that the photon flux is not limiting and back electron transfer is minimal, however the rate is significantly affected by formation of several reaction intermediates that are not photoactive. These thermal processes are very slow and control the overall rate. A design point for more efficient constructs would be to ensure all intermediates are photoactive.
2. CO2 reduction
Efficient (photo)electrochemical reduction of CO2 using scalable systems that convert solar energy into chemical energy holds promise as a sustainable way to generate low carbon fuels. Several of our projects in the Liquid Sunlight Alliance Energy Innovation Hub and under DOE Basic Energy Sciences funding use stochastic multiscale reaction-diffusion studies to examine key factors that affect the efficiency and the durability of these systems.
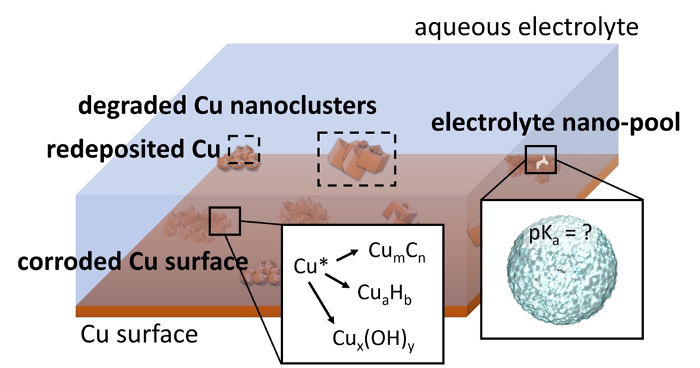
Nanoscale interactions involved in Cu corrosion
Team: Karnamohit Ranka, Richard Kang, Martin Head-Gordon (LBNL), Soonho Kwon, Prabhat Prakash, William Goddard (Caltech)
Copper cathode surfaces are the only ones that produce multicarbon products selectively. However, cathodic Cu corrosion competes with the reaction chemistry at Cu surfaces, continuously modifying the catalyst surface. Furthermore, the local chemical microenvironment at the Cu electrode-electrolyte interface is not completely understood, particularly in nanoscopic electrolyte pools in contact with the Cu surface often produced through trapping either inside pits of corroded Cu surfaces or by organic coatings on the Cu surface. We are building stochastic kinetic models to discover mechanisms responsible for cathodic Cu corrosion and gain insight into how fluctuations in the chemical composition of small pools of electrolyte influence reduction reactions. In past work we have studied water ion concentration fluctuations in pure water and are now investigating whether the size of the pool affects the dynamics of ion recombination and diffusion in order to determine which species are present near the Cu-electrolyte interface. We are also developing theoretical approaches necessary to obtain accurate electrochemical rate constants required for corrosion kinetics models.
Catalytic cascades on photoelectrodes
Team: Matthew Salazar (Caltech), Karnamohit Ranka, Peter Agbo, Sijia Ke, Jeff Neaton (LBNL), Emily Warren and Anne Greenaway (NREL)
A new tandem photoelectrode with 3 terminals can create separate regions with different potentials, allowing rates for steps in a catalytic cascade to be separately matched. We are developing models for these photoelectrodes that include full interfacial catalytic chemistry for Ag reacting with CO2 to form CO, and Cu reacting with both CO2 and CO to form desired products. As part of this work we are expanding how electrochemical rate constants can be calculated over a wide range of overpotentials, which will significantly extend the usefulness of theoretical estimates of reaction energetics for the many reaction steps involved in the catalysis.
Using signaling to regulate catalytic cascades – inspiration from natural systems
Team: Junko Yano, Peter Agbo, Philipp Simon, Joel Ager, Helen Zeng, Pan Wang
In this new project, we are developing experimental and computational approaches to use internal signaling to regulate catalytic cascade timing, as natural systems do. Signaling can involve changes in charge density, photoexcitations, and changes in local reactant concentrations. A combination of experiment and computation will enable us to design artificial systems that are as efficient and selective as natural ones.
II. Designing X-ray free electron laser experiments to measure protein reaction kinetics

Team: Casey Tsai, Alexis Esguerra, Daniel Ladiges, Philipp Simon, Hiroki Makita, Junko Yano and Jan Kern
The challenge to studying time-resolved protein reaction kinetics with selected substrates under ambient conditions is significant. X-ray free electron lasers open up this possibility because of their ultrafast intense pulses, but can require significant volumes of protein samples. The drop-on-drop-on-tape dispensing system is a recently developed instrument allows for collection of shot-by-shot diffraction patterns from individual drops while limiting sample consumption and sample damage. Under funding from NIH, a computational hydrodynamics/reaction-diffusion workflow is being developed to aid in design of the X-ray experiments and in interpretation of the data. This workflow is being tested by studying a proxy reaction with fluorescent dye and CaCl2 to ensure that the reaction conditions in the instrument are well-described. The X-ray experimental studies will involve slurries of protein crystallites, so we are also developing a protein permeation model to capture substrate uptake and reaction as droplets merge.
III. Nanoscale photolithography using EUV light.
Semiconductor manufacturing involves creating patterns in silicon wafers with high precision and high yield. These patterns are created using photolithography, where the key steps are exposure of a photoresist film to patterned light to create a latent image, heating to convert that image into a developable image, and dissolution of parts of the film to reveal the pattern. Research on how chemistry controls the quality of images created using 13.5 nm light, the current state of the art, is being carried out using DOE funding in the CHiPPS EFRC.
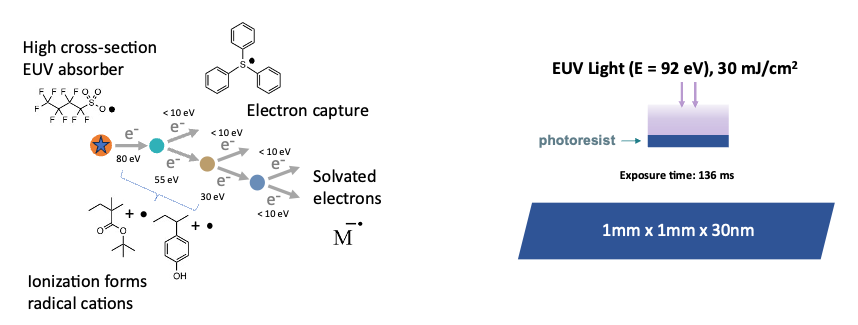
Imaging chemistry in organic photoresist materials
Team: Jacob Milton, Sam Blau, CHiPPS team members, and CRADA with Picarro Inc
The use of 13.5 nm light involves radiation chemistry leading to ionization and free radical formation, part of the imaging process. The mechanisms of these reactions in diverse resist types and how these reactions are connected to image formation and defects and imperfections in the patterns are poorly understood.
The complexity of the chemistry makes by-hand creation of proposed reaction mechanisms impossible. We have developed novel computational techniques to create a chemical reaction network that includes all energetically and chemically accessible steps that occur due to interaction with photons and high-energy electrons. This network uses thermodynamics calculated via high-throughput density functional theory workflows. The set of plausible reactions undergoes a huge number (~10,000) of kinetic Monte Carlo simulations to remove reactions that are kinetically infeasible based solely on the low concentrations of their reactants. Finally, this set of reactions (~5000) is imported into Kinetiscope, where we perform kinetic simulations with accurate populations and timescales. The simulation results are directly compared to experimental studies in CHiPPS to validate the chemical models for various materials compositions, and help interpret spectroscopic data.
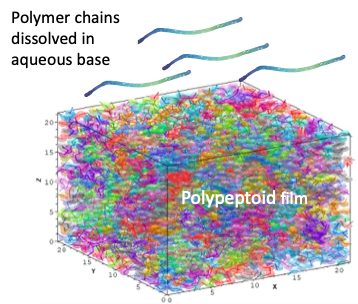
Experimental and modeling study of photoresist dissolution
Team: Jacob Milton (LBNL), Aung Paing, Kevin Zhao, Dahyun Oh (San Jose State University), Cameron Adams and Rachel Segalman (UCSB), William Hinsberg (Columbia Hill Technical Consulting)
A very successful model for resist dissolution during the pattern development step is the Critical Ionization Theory, which postulates that for a polymer chain in the resist to be able to dissolve in the basic developer solution, a critical fraction of the polymer chain’s OH groups need to be ionized. What this critical fraction is for various polymer compositions has not been rigorously established. Polypeptoid resists, which have a controlled sequence arrangement and chain length, resists offer a unique opportunity to establish what the critical fraction is for various polymer structures. We are measuring dissolution rates of pure polypeptoid materials using a quartz crystal microbalance and the ensuing resist roughness and simulating the dissolution process to test the Critical Ionization Theory’s predictions and establish the reaction rate constants that control solubility in imaged materials.